Intermittent fasting (IF) represents a family of eating regimens involving programmed periods of caloric restriction or complete abstention from food intake. A 2019 New England Journal of Medicine review by de Cabo and Mattson consolidates evidence connecting IF to neurological benefits, though most findings remain preliminary[1]. This article examines the molecular pathways potentially underlying these benefits, distinguishing established mechanisms from speculative ones.
Defining Clinical Intermittent Fasting Protocols
Four primary IF approaches have emerged in clinical research:
- Time-Restricted Eating (TRE): Daily food consumption confined to 8-12 hours. A 2015 review by Tinsley and La Bounty found this method particularly sustainable for adherence due to its daily consistency[2]. The 16/8 protocol (16 hours fasting, 8 hours eating) represents the most studied variant. Notably, Patterson et al. observed in their 2015 analysis that aligning eating windows with circadian rhythms may enhance metabolic benefits[17].
- Alternate-Day Fasting (ADF): Rotation between normal-calorie days and either severe reduction days (≤25% normal intake) or complete fasting days. Varady's 2022 clinical review confirmed efficacy for weight loss comparable to continuous restriction but with greater adherence in certain populations[3]. Their research documented mean weight reductions of 3-8% after 2-3 months across multiple clinical trials.
- 5:2 Diet: Normal eating five days weekly with two non-consecutive ~500-600 kcal days. Originally popularized through clinical work at the University of Manchester, this protocol offers weekend flexibility valued by participants.
- Periodic Prolonged Fasting: Fasting periods exceeding 24 hours, implemented weekly or monthly. This approach most closely resembles the evolutionary fasting patterns studied in anthropological research but presents adherence challenges in modern settings.
The Neurometabolic Switch: Ketones as Brain Fuel and Signaling Molecules
Anton et al. coined the term "metabolic switch" in their 2018 review to describe the body's transition from glucose to ketone utilization[4]. This process occurs approximately 12-36 hours after fasting onset, when liver glycogen stores deplete, as documented in Cahill's seminal starvation metabolism studies[6].
The brain's relationship with ketones extends beyond simple fuel substitution. In a landmark 2018 Nature Reviews Neuroscience paper, Mattson and colleagues identified three key aspects of ketone metabolism in neural tissue[5]:
- Enhanced Mitochondrial Efficiency: Neurons utilize beta-hydroxybutyrate (BHB) with greater ATP yield per oxygen molecule compared to glucose, potentially reducing oxidative stress.
- Epigenetic Regulation: BHB functions as a histone deacetylase inhibitor, modifying gene expression patterns that promote neural resilience and plasticity.
- Anti-inflammatory Signaling: Ketones activate hydroxycarboxylic acid receptor 2 (HCA2), suppressing microglial activation and neuroinflammation.
A critical distinction made by Longo and Mattson in their 2014 Cell Metabolism review is that neurological benefits may require higher ketone thresholds than metabolic improvements[18]. Blood BHB levels typically reach 0.5-1 mM after 16-24 hours of fasting but may need to exceed 1-2 mM to fully engage neuroprotective pathways.
Fasting Metabolic Phases and Brain Effects
Phase | Timing | Metabolic State | Neural Effects |
---|---|---|---|
Fed/Absorptive | 0-4 hours after meal | Glucose primary fuel; insulin elevated | Baseline activity; anabolic processes |
Postabsorptive | 4-16 hours | Liver glycogen utilization; glucagon rises | Early stress response activation; FOXO signaling |
Early Fasting | 16-24 hours | Lipolysis increases; ketogenesis begins | Autophagy induction; antioxidant upregulation |
Ketotic | 24-72+ hours | Full ketosis; protein sparing | BDNF elevation; anti-inflammatory effects; neurogenesis support |
Adapted from metabolic phase data from Cahill (2006)[6] and neural effects from Mattson et al. (2018)[5]
Hormetic Stress Responses and Neuroprotective Adaptations
A comprehensive 2017 review in Aging Research Reviews by Mattson and colleagues identified cellular stress response mechanisms activated by IF[8]. These exemplify hormesis—beneficial biological adaptations triggered by mild stressors. Key mechanisms include:
- Enhanced Antioxidant Defenses: IF upregulates nuclear factor erythroid 2-related factor 2 (Nrf2), the master regulator of cellular redox homeostasis. Nrf2 activation increases transcription of enzymes including superoxide dismutase and glutathione peroxidase, bolstering neural resilience against oxidative damage.
- Mitochondrial Adaptation: Fasting periods stimulate mitochondrial biogenesis through peroxisome proliferator-activated receptor gamma coactivator 1-alpha (PGC-1α) and improves respiratory efficiency. Mitochondrial networks undergo dynamic remodeling favoring fusion over fission, enhancing functional capacity.
- Autophagy Activation: Nutrient sensing pathways, particularly reduced mechanistic target of rapamycin (mTOR) signaling during fasting, trigger autophagy—the cellular process removing damaged organelles and protein aggregates. This "quality control" mechanism is particularly crucial for post-mitotic neurons vulnerable to protein accumulation pathologies.
- Neurotrophic Factor Induction: Rodent studies consistently demonstrate increased brain-derived neurotrophic factor (BDNF) expression in the hippocampus, cortex, and striatum following intermittent fasting. BDNF enhances synaptic plasticity, neurogenesis, and cellular stress resistance—effects potentially linked to cognitive enhancement.
De Cabo and Mattson's 2019 review emphasizes an important limitation: while these mechanisms are well-documented in animal models, human evidence remains largely inferential[1]. Translational challenges include species differences in fasting metabolism, limited brain tissue accessibility in humans, and ethical constraints on experimental protocols.
Research Spotlight: Mattson's Neuroplasticity Findings
Neuroscientist Mark Mattson's laboratory at the National Institute on Aging has conducted numerous studies examining the neuroplastic effects of intermittent fasting. In a pivotal 2018 review in Nature Reviews Neuroscience[5], Mattson summarized research showing IF enhanced hippocampal neurogenesis in rodents while increasing synthesis of BDNF and neurotrophic growth factor. These changes correlated with improved performance on spatial and contextual memory tasks. Particularly intriguing was the finding that these benefits persisted even when caloric intake matched control animals, suggesting timing of food intake—not merely total calories—influences brain adaptation.
Neuropsychiatric Effects: Mood, Cognition, and Clinical Implications
The relationship between fasting and neuropsychiatric function shows bidirectional effects. Fond et al. published a systematic review in 2013 examining mood effects of fasting, finding improvements in depression and anxiety scales among participants in prolonged fasting programs[9]. The authors proposed several potential mechanisms:
- Ketone body-mediated modulation of neurotransmitter systems, particularly GABA and glutamate balance
- Enhanced endogenous endocannabinoid signaling as adipose tissue releases stored lipids
- Reset of circadian gene expression patterns influencing mood regulation
Similarly, a 2010 review by Michalsen in Current Pain and Headache Reports documented mood enhancement in patients with chronic pain syndromes who underwent therapeutic fasting[10]. Counter-intuitively, participants reported decreased pain perception and improved mood despite the expected stress of food deprivation.
However, mood effects depend significantly on timeframe. Horne and colleagues' 2015 systematic review noted that early adaptation to fasting (first 1-3 days) frequently involves negative symptoms including irritability, concentration difficulties, and headaches[11]. These transient effects typically diminish with continued practice or disappear entirely after 3-4 weeks of consistent fasting routines.
Regarding cognitive function, current evidence remains mixed and context-dependent. Acute fasting appears to enhance some aspects of cognition (attention, executive function) while impairing others (working memory, processing speed), with effects highly dependent on baseline metabolic health, age, and fasting duration. Notably, studies typically show greater cognitive benefits in participants with metabolic dysfunction at baseline than in already-healthy subjects.
Safety Profile and Clinical Considerations
A 2022 Nature Reviews Endocrinology analysis by Varady et al. established safety profiles for various IF protocols[3]. In healthy overweight adults, TRE and modified ADF demonstrate good safety and tolerability profiles in trials up to one year. Transient side effects typically resolve within the first month and include:
- Hunger and occasional irritability
- Mild headaches, particularly during initial adaptation
- Light-headedness or dizziness (less common)
- Constipation (observed in some protocols)
Several major health authorities have published specific contraindications. The National Institute on Aging explicitly advises against IF for specific populations[12]:
- Children and adolescents (potential interference with growth)
- Pregnant or breastfeeding women
- Individuals with BMI under 18.5
- Those with current or historical eating disorders
- Adults over 75 without close medical supervision
For individuals with type 1 or type 2 diabetes, fasting presents special considerations. The American Diabetes Association's 2019 consensus report, authored by Evert et al., emphasizes the substantial risk of hypoglycemia during fasting, particularly for those using insulin or secretagogues[15]. They recommend mandatory medical supervision with medication adjustments and glucose monitoring protocols if IF is attempted by diabetic patients.
Persynaki and colleagues' 2017 analysis of religious fasting provides additional insights into safety considerations based on centuries of observational data[14]. Their work highlights that even in healthy individuals, extreme environmental conditions (heat, physical exertion) significantly increase risks during fasting periods.
Emerging Research Directions
Several critical questions remain actively investigated in the IF-brain research domain:
- Neuroprotection in Neurodegenerative Disease: Animal models show promising neuroprotective effects in Alzheimer's, Parkinson's, and Huntington's disease models, but human clinical trials remain preliminary.
- Circadian Alignment: Growing evidence suggests meal timing relative to circadian rhythms significantly influences metabolic outcomes. Early time-restricted eating (eTRE), confining food intake to earlier daylight hours, appears particularly beneficial.
- Individual Response Variation: Genetic polymorphisms affecting metabotype, gut microbiome composition, and baseline metabolic health create substantial variation in fasting responses. Personalized approaches may be necessary to optimize outcomes.
- Combined Interventions: Synergistic effects between IF and other interventions (exercise, ketogenic diet, specific nutrients) represent a promising frontier for enhanced neurological benefits.
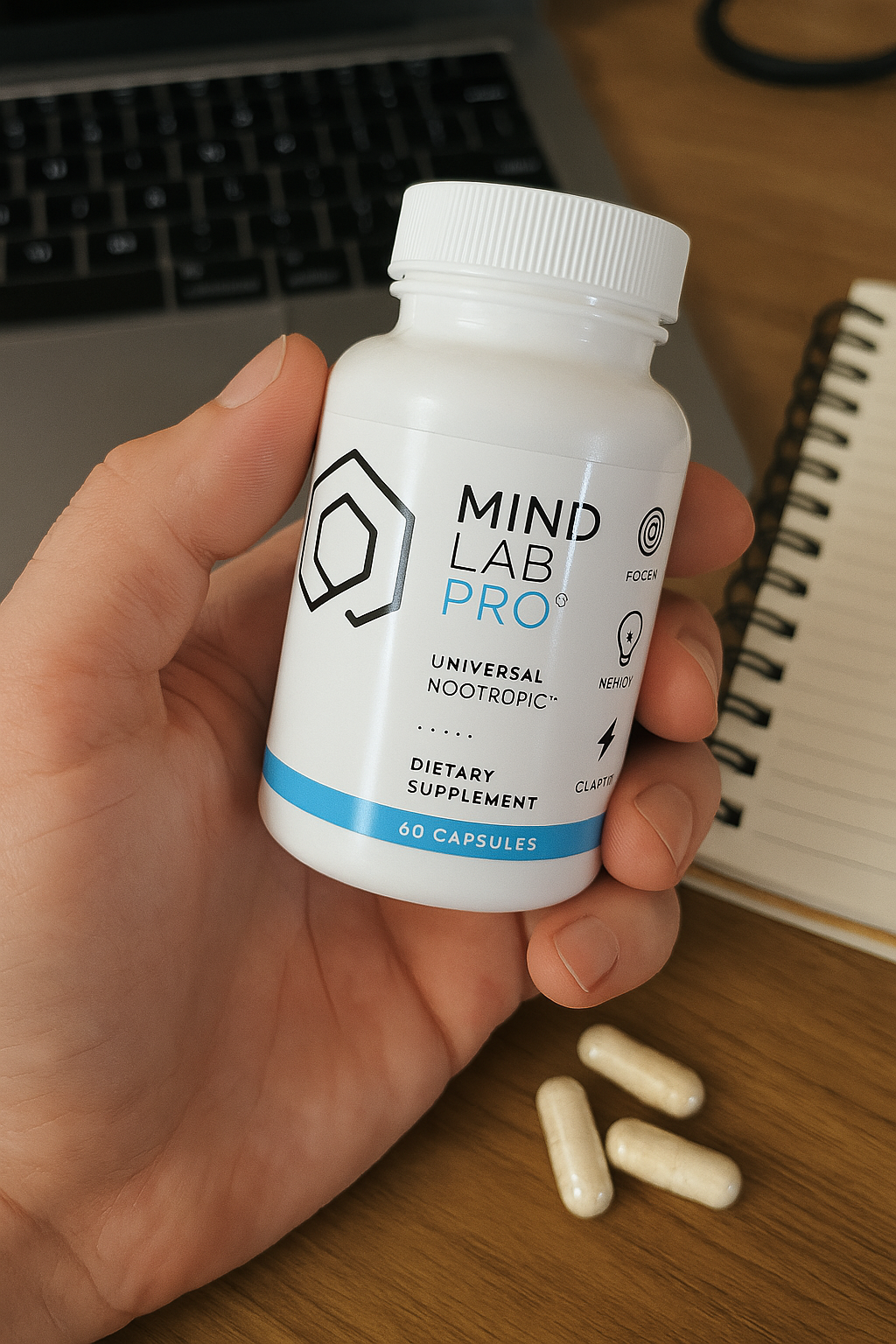
Mind Lab Pro
- Complete 11-in-1 formula
- Non-stimulant formula
- Premium price
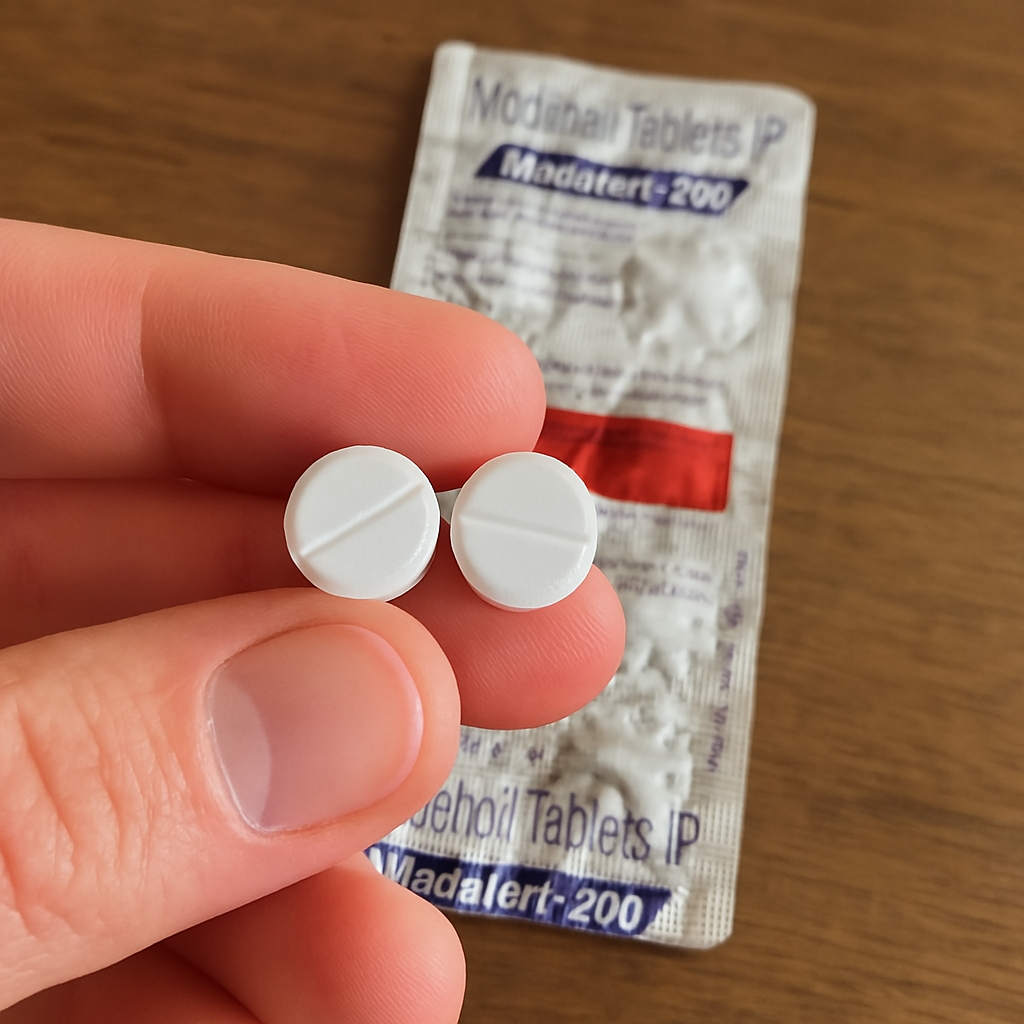
Modafinil
- Powerful focus
- 12+ hours duration
- Rx required in US
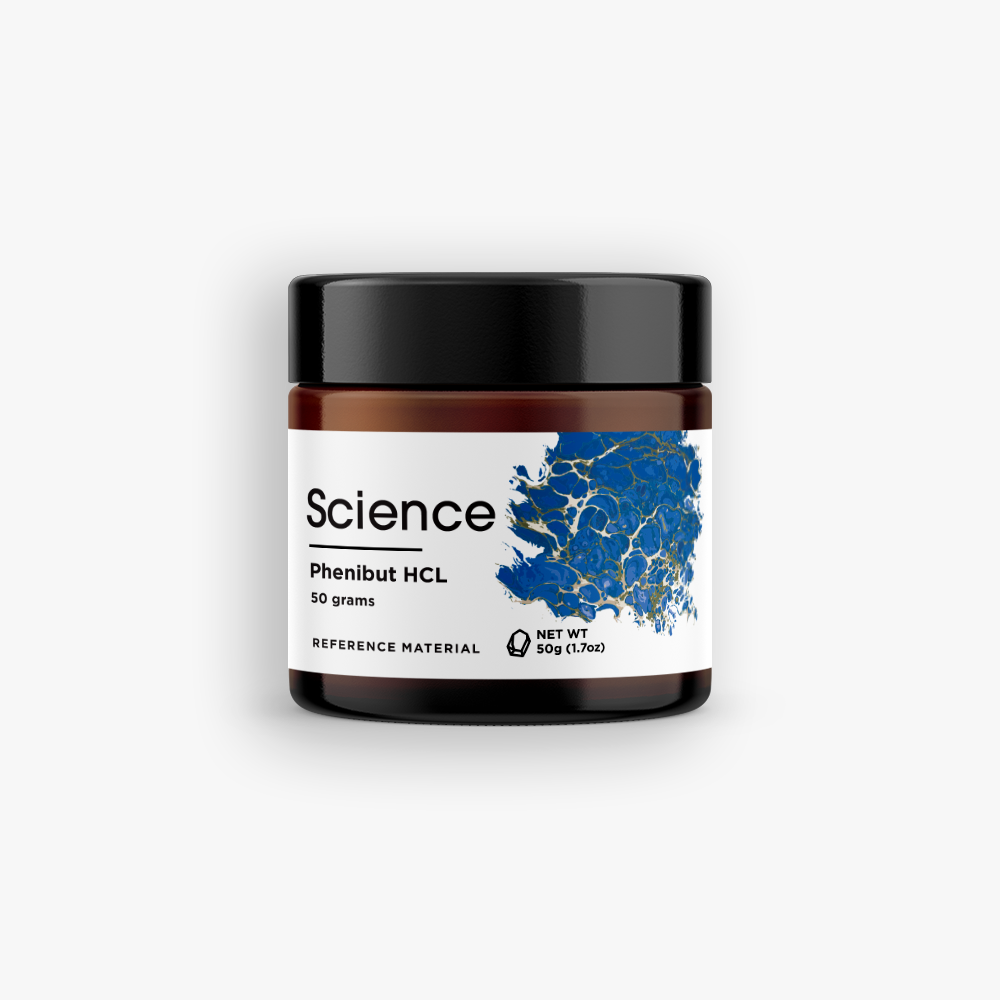
Science.bio
- Third-party tested
- Many compounds
- Research only
Conclusion: Evidence-Based Perspective
The current state of evidence suggests IF triggers neurologically relevant metabolic and cellular adaptations that could feasibly enhance brain health. Mechanistic pathways involving ketone metabolism, stress resistance signaling, and improved mitochondrial function provide a plausible biological basis for observed benefits in preclinical models.
However, translation to definitive human neurological benefits remains incompletely demonstrated. While meta-analyses like Cioffi et al.'s 2018 review confirm metabolic improvements[16], direct evidence for cognitive enhancement, mood stabilization, or neuroprotection in humans lacks the robustness of the mechanistic foundation.
Individuals considering IF for brain health should maintain realistic expectations aligned with current science: IF represents a promising but still experimental approach for neurological benefits. Implementation warrants caution, appropriate medical consultation, and self-monitoring, particularly for those with pre-existing health conditions. As research advances, the specific protocols, populations, and adjunctive measures most likely to enhance neurological outcomes will become clearer.
References
- de Cabo R, Mattson MP. "Effects of Intermittent Fasting on Health, Aging, and Disease." NEJM. 2019. (source)
- Tinsley GM, La Bounty PM. "Effects of intermittent fasting on body composition and clinical health markers in humans." Nutr Rev. 2015. (source)
- Varady KA, et al. "Clinical application of intermittent fasting for weight loss..." Nat Rev Endocrinol. 2022. (source)
- Anton SD, et al. "Flipping the Metabolic Switch: Understanding and Applying the Health Benefits of Fasting." Obesity. 2018. (source)
- Mattson MP, et al. "Intermittent metabolic switching, neuroplasticity and brain health." Nat Rev Neurosci. 2018. (source)
- Cahill GF. "Fuel metabolism in starvation." Annu Rev Nutr. 2006. (source)
- Drăgoi CM. "Insights into Chrononutrition..." Farmacia. 2019. (source)
- Mattson MP, et al. "Impact of intermittent fasting on health and disease processes." Ageing Res Rev. 2017. (source)
- Fond G, et al. "Fasting in mood disorders: neurobiology and effectiveness." Psychiatry Res. 2013. (source)
- Michalsen A. "Prolonged fasting as a method of mood enhancement in chronic pain syndromes..." Curr Pain Headache Rep. 2010. (source)
- Horne BD, et al. "Health effects of intermittent fasting: hormesis or harm? A systematic review." Am J Clin Nutr. 2015. (source)
- National Institute on Aging. "Calorie restriction and fasting diets: What do we know?" 2018. (source)
- "Diet Review: Intermittent Fasting for Weight Loss". Harvard School of Public Health. 2018. (source)
- Persynaki A, et al. "Unraveling the metabolic health benefits of fasting related to religious beliefs..." Nutrition. 2017. (source)
- Evert AB, et al. "Nutrition Therapy for Adults With Diabetes or Prediabetes: A Consensus Report." Diabetes Care. 2019. (source)
- Cioffi I, et al. "Intermittent versus continuous energy restriction on weight loss and cardiometabolic outcomes..." J Transl Med. 2018. (source)
- Patterson RE, et al. "Intermittent Fasting and Human Metabolic Health." J Acad Nutr Diet. 2015. (source)
- Longo VD, Mattson MP. "Fasting: molecular mechanisms and clinical applications." Cell Metab. 2014. (source)
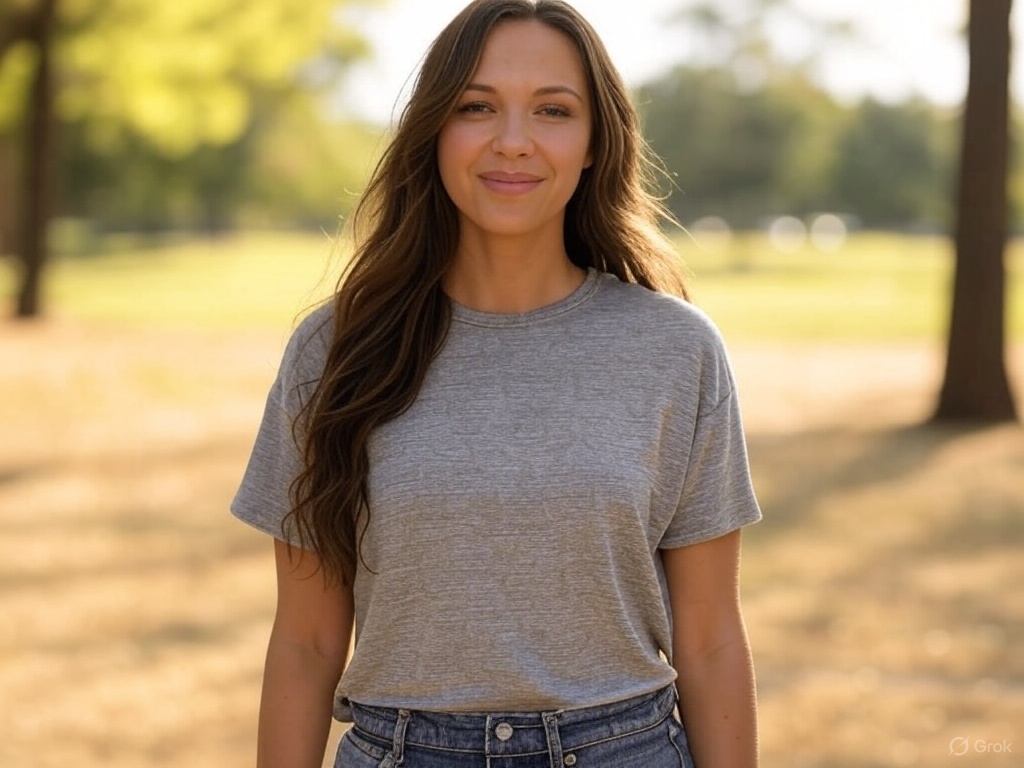
Jen Masson
Brain Nutrition Specialist with expertise in nootropics, ketogenic diets, cognitive and metabolic optimization.